What Happened to the Nuclear Renaissance?
What Happened to the Nuclear Renaissance?
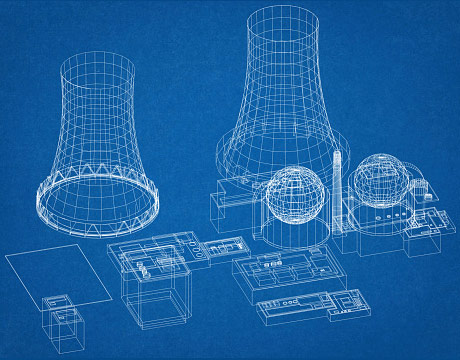
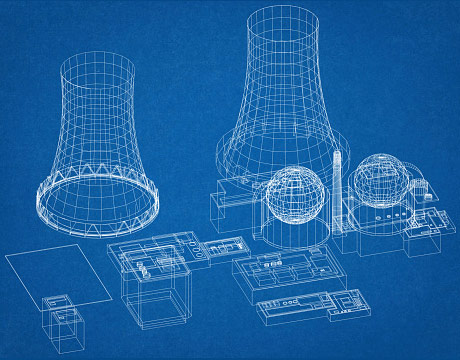
The industry must embrace new ideas and develop safe, simple, and cost-competitive reactors before a true “Nuclear Renaissance” can occur.
We should be living in the best of times for the nuclear power industry. Nuclear power has marked advantages over competing power sources. It produces almost no net carbon emissions, unlike natural gas, oil, or coal, and it is more or less “always on,” operating at several times higher capacity factor than wind or solar power. Nuclear plants can be sited in a wide variety of locations, unlike hydroelectric dams, and have small footprints. They are well suited to enable such innovations as the electrification of transportation or hydrogen co-generation.
What’s more, interest rates have been quite low for more than a decade, making financing large capital projects more affordable—crucial for nuclear power, which has low operating costs but large upfront costs.
Finally, nuclear power has benefited from the political, technical, and often financial support of governments around the world as they seek energy security and diversity of supply. To be sure, not every government has been supportive—Germany, for one, has moved to close its nuclear fleet—but generally governments have provided research and development money, tax breaks, or even loans for construction funding depending on the local political situation.
In the early 2000s, the talk was of a so-called Nuclear Renaissance with hundreds of new nuclear plants to be ordered. We should be seeing the fruits of that now, but we are not.
Yes, there has been interest and expansion in the nuclear industry in developing or “emerging” nations, including China, Russia, India, Brazil, and the United Arab Emirates. And even in the United States, improved operations has led to an increase in net generation—power from nuclear plants surpassed that from coal plants for the month of April 2019.
On the whole, however, the nuclear industry in the traditionally industrialized world has been marked by stagnation and reversal, rather than renaissance. Few new plants are being built and existing plants are being retired.
The International Atomic Energy Agency (IAEA), looking ahead to 2050, sees the most optimistic global electricity market share for nuclear as only about 5 percent, down from 10 percent today; and in the United States and Europe, it steadily declines to between 3 and 5 percent of the market, constituting potential for market “failure.”
The obvious—and completely undeniable—factors that have led to this stagnation are:
• The aftermath of the Chernobyl and Fukushima reactor accidents. The problem wasn’t so much the damage from those accidents per se, but the sheer cost, which was enough to bankrupt even the most deep-pocketed owner or operator. Public panic has resulted in increased licensing times, design costs, and provision of extra backup power and cooling options for existing and new plants.
• The massive rise of unconventional oil and gas production. Thanks to “fracking,” the United States is producing and even exporting low-cost fossil fuels. This has undermined worldwide nuclear industry sales by rendering nuclear plants noncompetitive in open power markets, where such less expensive alternatives are now available. Indeed, switching to gas has reduced emissions, lowered generating costs, and increased efficiency compared to coal.
• Loss of confidence in project completion and costs. Difficulties with large nuclear projects have led to the bankruptcy or reorganization of three major vendors—Westinghouse in the U.S., Areva in France, and AECL in Canada. Now, financial risk premiums in investment markets favor short-term guaranteed returns and low unknown risks, which current or projected nuclear projects cannot provide without subsidies or financial support guarantees, including enforced power purchase agreements and prices.
Further Reading: Storing Heat from Nuclear Power Plants Could Improve Output
This state of affairs matters beyond the stakeholders of the nuclear industry. A program of building thousands of 1,000-MWe scale reactors worldwide in the coming decades is perhaps the best path toward meeting projected economic and energy demands, while assisting in stabilizing global carbon dioxide emissions.
To revive the sector so it can meet that challenge, it is important to evaluate the current status of the nuclear power industry, examine the requirements that all new power technologies in open and competitive markets must meet, and determine whether present and future nuclear-power technologies look to be capable of achieving those goals.
Since the Fukushima nuclear accident in March 2011, nuclear’s share of global electricity production has dropped from 14 percent to around 10 percent today, even while net generation has increased by operating the existing units longer and harder.
Older units are being retired and the pace of new nuclear-reactor construction is glacial (except in China) such that the net number of operating reactors worldwide increased by only five from 2011 to 2019. In many markets, the decline in coal power due to concerns over carbon pollution has been met by an increase in combustion turbines fueled by cheap natural gas, or by wind turbines often subsidized by government tax credits, renewable portfolios, and mandatory feed-in-tariffs. Neither of those sources have the advantages of nuclear’s low emissions, high capacity factors, and long life.
Currently, 31 countries in the world have operating nuclear-power reactors, with a total installed capacity of about 400 GW, and since March 2011, the net operating reactor capacity has increased by 23 GW. That rough stability hides quite a bit of churn, as China added 34 reactors over that period while Germany, Japan, the United Kingdom, and the United States cumulatively reduced their number by 37.
Looking ahead, 18 of the countries currently operating nuclear-power reactors plan to add new units, as do five countries (Bangladesh, Belarus, Egypt, Turkey, and the United Arab Emirates) planning their first reactors.
New build activity is dominated by state-supported manufacturers from China, Russia, and South Korea with either financing or political guarantees; vendors from those three countries are building 60 new reactors around the world.
In more open markets, there are techniques to encourage and guarantee investor returns, all of which mean the customer pays for the plant one way or another. Those market-oriented approaches include a variety of pricing mechanisms and a “regulated asset base model,” where a regulator would estimate a value of new nuclear projects and arrive at a fixed rate of return for investors, with consumers paying upfront through electricity bills.
Related Video: Nuclear Power: Safety and Reliability
The reason for these sorts of financing mechanisms is simple. Recently completed projects and possible nuclear designs are more expensive than alternatives, such as those that burn natural gas.
Also, with new capacity added in a few, very large increments and suppliers scattered throughout the world, it is difficult to build and sustain manufacturing and supply chain capability. That limits the ability to mass produce multiple units of the same design. Mass production is further hampered by the lack of an agreed international licensing process or “certification” for essentially duplicate designs, as adopted in and by the airline industry.
There is also a scarcity of global investment in R&D and demonstrations of completely new technologies, except in China, where literally “one-of-every-type” is being built and tested.
When the worldwide nuclear building plans are totaled, the numbers are promising: about 90 reactors and more than 90,000 MWe of net electrical output are slated to be built in the coming decade. Whether the nuclear power industry can meet this building schedule—or even keep the net number of operating reactors steady—remains to be seen. But the world needs even more.
One potential way to break out of the current stasis is to develop a new generation of nuclear technology.
To be sure, many companies and organizations are working on concepts that are on a different scale, or use different fuel or alternative cooling media, or deviate in some other fundamental way from the light water reactors that dominate the industry at present.
Assessing the prospects of these concepts in not always easy. The key to the successful deployment of any such new or next-generation nuclear concept or design is the ability to compete against available energy alternates, especially, in local or national power markets.
The competition isn’t only the economics of supplying power, however, but rather holistic.
How should these proposals be assessed? This is not rocket science, but does require objective and rational thinking.
The requirements and internal challenges for any new nuclear concept, design, or technology can be summarized as follows:
• They should be safer than previous “generations” of nuclear technology;
• They should require a low financial risk exposure and capital cost;
• They should be easy to build quickly;
• They should be readily licensable by national authorities;
• They should be secure and simple to operate;
• They should have an assured and sustainable fuel supply;
• They should provide social value and acceptance;
• They should be competitive with respect to lowest cost generation.
One key factor that these requirements have in common is that they are quiet, if not exactly silent, on technological requirements. As long as a reactor concept can meet these eight challenges—meaning that it is “better” than existing nuclear power plants as well as gas turbines and coal thermal plants—it doesn’t matter what the fuel is or how it is cooled.
Further Reading: Harvesting the Power of the Ocean
It is the market that sets the needs and the requirements, and the exact design or means of achieving the breakthrough is secondary.
In any event, the reactor island is a small fraction of the total plant or project costs, so it is evident that technology choice is not crucial. The real issue is fully optimizing the overall cost and efficiency of the design and performance of any new technology to meet power- and financial-market requirements, not choosing or developing something that is superficially attractive, but too expensive.
For all the current advances in nuclear power, nuclear power plants have the following deficiencies: They generate radioactive wastes; they have relatively low thermal efficiencies, especially those plants equipped with water-cooled reactors; they risk radiation release during severe accidents; and they rely on nuclear fuel produced through environment-unfriendly processes. None of that is acceptable.
To expand the share of electricity produced by nuclear power—or to simply forestall a decline in nuclear’s “market share”—will require addressing those deficiencies as well as the eight requirements listed earlier.
A way to insure this is to establish the sales potential, target markets, and performance goals prior to committing to the preliminary design work. That can be done iteratively, by first assessing the optimum capital, operating, and generating costs as a function of plant output size to determine the system design targets and technical requirements. After that, one would need to establish investment needs and suitable power purchase agreements or contracts. Finally, one can determine the build profile and output that matches the power market and customer generating needs, iterating through the previous steps as needed, and if necessary changing or even adopting a different technology.
This systematic method provides a coherent business model for both supplier and customer, and is also useful as a rapid audit and estimating tool, and to weed out uncompetitive options.
Licensing, siting, fuel, and decommissioning costs are difficult to reduce substantially, so to meet the market needs, capital- and operating-cost reduction is the obvious first target. This argues against large plants, as recent build experience in China, Europe, and the United States shows that large plants often require more than 60 months to complete or experience significant delays in construction, and long schedules and delays are the major factor that must be avoided, since the levelized cost of energy grows almost linearly with the increase in project timescale.
Instead, the oft-stated objectives are to simplify and “modularize” designs, reducing capital and operating costs, and shortening construction times, perhaps building multiple smaller units instead of one large one to spread the risk and costs.
From Our Archives: Chernobyl 25 Years Later
The fundamental problem is that a decrease in plant output increases the levelized cost of energy, because many of the balance of plant and other variable and fixed costs (such as site, safety, infrastructure, engineering, decommissioning, and staffing) do not decrease proportionately, so ultimately become dominant as output shrinks. Also, unless there is a commitment to build a substantial number of these simplified, production-line or “Henry Ford” reactors, the engineering, design, licensing, and set-up costs all have to be absorbed by the first few units, increasing the cost of the first-of-its-kind unit far above the economic break-even point.
Instead, the nuclear industry could take a chapter from the aircraft manufacturing industry and use the “order book” approach, in which a company receives a commitment from customers for some set number of units of the same kind before it initiates a building program. To “fill the order book” is design and market specific, but the cost reduction possible from mass production is able to offset the penalty from building smaller units and plants.
(While both theory and real-world production data suggests that producing 10 or more units should decrease costs by up to 50 percent, it is now known that the actual AP1000 costs in the United States do not follow those projections, and neither do the current EPR builds in France and Finland, primarily due to long delays in construction and project schedule increasing the costs.)
From Our Archives: After Fukushima, ASME Task Force Challenges Nuclear Industry
Another, related concept is modular construction, which is widely used in oil rigs, military equipment, buildings, data centers, computers, and combined-cycle gas turbine installations. Rather than single large units, modules would be smaller in scale and could be added as needed to meet the local market demand for electricity.
Even the systems that make up the larger module can be “sub-modularized,” especially important when redundancy is needed for safety, maintenance, and performance reasons. The small modular reactor concept, or SMR, is predicated on each reactor unit being built in a factory of sorts and shipped to the site, rather than constructed on the spot.
Investment in module “factories” is expensive, requires large upfront commitment (for say, options for 100 standardized units in the “order book”), and the downside risks must be carefully managed, since that cost must also be subtracted, or amortized over the sale of many units. It is self-evident that although small units cost more for their power and energy, when mass produced they carry significantly less financial risk and for much shorter exposure times.
It is undeniable that SMRs are a very “hot” topic in nuclear engineering worldwide. Smaller or even “six pack” units could reduce investment risk, while adding capacity in increments to penetrate smaller electric grids and markets. According to the IAEA, there are about 55 SMRs designs or concepts proposed in the world. In 2019, Russia launched and licensed Akademik Lomonosov, a barge carrying its first two SMRs-KLT-40S reactors, intended as a floating nuclear power plant supplying 70 MW of electricity and heat for the country’s northern regions.
While the SMR concept is appealing, in fact, recent comparative studies by the Nuclear Energy Agency and the Nuclear Fuel Cycle Royal Commission in South Australia failed to demonstrate a clear market or price advantage for current SMR concepts, compared to competing nuclear technologies.
The challenges facing the nuclear industry as a whole—such as the low capital cost and high efficiency of modern natural gas and supercritical-pressure coal-fired power plants—will likely weigh down the development and deployment of SMRs in the near term.
A simple comparison of recently published or deduced actual costs shows that presently built or proposed multi-unit nuclear plants (both large and small) must reduce specific capital and generating costs per kW by some 20 to 40 percent to compete with today’s market-ready combined-cycle gas turbines (CCGTs). This is not an easy target, and requires some radical rethinking of today’s developments—and discarding some concepts.
The next generation of nuclear power plants should be based on new designs—not just old ideas that have been rehashed yet again—to achieve the eight requirements. And these new designs must be better than “safe enough” or “safer than coal”; they should feature walk-away safety that precludes large accidents, core meltdown, and possible radiation releases, as well as the potential for weapons proliferation. The designs must produce electricity as cheaply as natural gas and with an efficiency in the range of CCGTs—better than 55 percent. Any new design must have broad public and regulatory acceptance and be capable of rapid licensing.
And it should go without saying that the next generation of nuclear reactors must provide for real, worldwide carbon-dioxide reduction without needing “carbon credits” or “offsets” to compete.
Further Reading: Startup Suns
For new nuclear power to contribute meaningfully to the fight against global warming requires a building program on a massive scale: Thousands of new units would need to come online, better than one per week from now until 2050. The industry is nowhere close to meeting that challenge, and policy makers nowhere near to accepting it—although the political realization is slowly growing that nuclear energy is necessary to the energy and environmental future of the planet.
The nuclear industry will need to take a hardheaded approach to get there. It must avoid fads and fantasies, as well as the lazy assertions that the world has no other options. It will require both the will to act and money in amounts large enough to turn the will into action. Nonprofit funding from groups such as the Gates Foundation is welcome, but not sufficient; it will require both private-sector and government investment.
Innovation is key, as is a willingness to reassess the assumptions of the past. Obviously, to meet the challenges set before the industry will require new directions in reactor design. But it also requires producing reactors that can match the needs of many different markets, both in scale and in cost. Small modular reactors (whatever that ultimately means) are one way to flexibly address the needs of markets, but it isn’t the only way, and it may not be appropriate for some markets. The tendency toward one-size-fits-all solutions is a poor one, regardless of the size.
To revive the nuclear-power sector will require not only a change in technology but also in mindset. It is too strong to say that the industry will have to start from scratch—after many decades, there is much to build on—but it is fair to say that a successful nuclear sector in 2050 will be radically transformed from what it is today.
Romney Duffey is former chair of ASME Nuclear Engineering Division and former principal scientist for Atomic Energy of Canada Limited. Igor Pioro is a professor of energy systems and nuclear science at the University of Ontario Institute of Technology in Oshawa. Pioro is the founding editor of the ASME Journal of Nuclear Engineering and Radiation Science.
This article is based on a JNERS paper from April 2019.
What’s more, interest rates have been quite low for more than a decade, making financing large capital projects more affordable—crucial for nuclear power, which has low operating costs but large upfront costs.
Finally, nuclear power has benefited from the political, technical, and often financial support of governments around the world as they seek energy security and diversity of supply. To be sure, not every government has been supportive—Germany, for one, has moved to close its nuclear fleet—but generally governments have provided research and development money, tax breaks, or even loans for construction funding depending on the local political situation.
In the early 2000s, the talk was of a so-called Nuclear Renaissance with hundreds of new nuclear plants to be ordered. We should be seeing the fruits of that now, but we are not.
Yes, there has been interest and expansion in the nuclear industry in developing or “emerging” nations, including China, Russia, India, Brazil, and the United Arab Emirates. And even in the United States, improved operations has led to an increase in net generation—power from nuclear plants surpassed that from coal plants for the month of April 2019.
On the whole, however, the nuclear industry in the traditionally industrialized world has been marked by stagnation and reversal, rather than renaissance. Few new plants are being built and existing plants are being retired.
The State of Affairs
The International Atomic Energy Agency (IAEA), looking ahead to 2050, sees the most optimistic global electricity market share for nuclear as only about 5 percent, down from 10 percent today; and in the United States and Europe, it steadily declines to between 3 and 5 percent of the market, constituting potential for market “failure.”
The obvious—and completely undeniable—factors that have led to this stagnation are:
• The aftermath of the Chernobyl and Fukushima reactor accidents. The problem wasn’t so much the damage from those accidents per se, but the sheer cost, which was enough to bankrupt even the most deep-pocketed owner or operator. Public panic has resulted in increased licensing times, design costs, and provision of extra backup power and cooling options for existing and new plants.
• The massive rise of unconventional oil and gas production. Thanks to “fracking,” the United States is producing and even exporting low-cost fossil fuels. This has undermined worldwide nuclear industry sales by rendering nuclear plants noncompetitive in open power markets, where such less expensive alternatives are now available. Indeed, switching to gas has reduced emissions, lowered generating costs, and increased efficiency compared to coal.
• Loss of confidence in project completion and costs. Difficulties with large nuclear projects have led to the bankruptcy or reorganization of three major vendors—Westinghouse in the U.S., Areva in France, and AECL in Canada. Now, financial risk premiums in investment markets favor short-term guaranteed returns and low unknown risks, which current or projected nuclear projects cannot provide without subsidies or financial support guarantees, including enforced power purchase agreements and prices.
Further Reading: Storing Heat from Nuclear Power Plants Could Improve Output
This state of affairs matters beyond the stakeholders of the nuclear industry. A program of building thousands of 1,000-MWe scale reactors worldwide in the coming decades is perhaps the best path toward meeting projected economic and energy demands, while assisting in stabilizing global carbon dioxide emissions.
To revive the sector so it can meet that challenge, it is important to evaluate the current status of the nuclear power industry, examine the requirements that all new power technologies in open and competitive markets must meet, and determine whether present and future nuclear-power technologies look to be capable of achieving those goals.
Since the Fukushima nuclear accident in March 2011, nuclear’s share of global electricity production has dropped from 14 percent to around 10 percent today, even while net generation has increased by operating the existing units longer and harder.
Older units are being retired and the pace of new nuclear-reactor construction is glacial (except in China) such that the net number of operating reactors worldwide increased by only five from 2011 to 2019. In many markets, the decline in coal power due to concerns over carbon pollution has been met by an increase in combustion turbines fueled by cheap natural gas, or by wind turbines often subsidized by government tax credits, renewable portfolios, and mandatory feed-in-tariffs. Neither of those sources have the advantages of nuclear’s low emissions, high capacity factors, and long life.
Currently, 31 countries in the world have operating nuclear-power reactors, with a total installed capacity of about 400 GW, and since March 2011, the net operating reactor capacity has increased by 23 GW. That rough stability hides quite a bit of churn, as China added 34 reactors over that period while Germany, Japan, the United Kingdom, and the United States cumulatively reduced their number by 37.
Future Plans
Looking ahead, 18 of the countries currently operating nuclear-power reactors plan to add new units, as do five countries (Bangladesh, Belarus, Egypt, Turkey, and the United Arab Emirates) planning their first reactors.
New build activity is dominated by state-supported manufacturers from China, Russia, and South Korea with either financing or political guarantees; vendors from those three countries are building 60 new reactors around the world.
In more open markets, there are techniques to encourage and guarantee investor returns, all of which mean the customer pays for the plant one way or another. Those market-oriented approaches include a variety of pricing mechanisms and a “regulated asset base model,” where a regulator would estimate a value of new nuclear projects and arrive at a fixed rate of return for investors, with consumers paying upfront through electricity bills.
Related Video: Nuclear Power: Safety and Reliability
The reason for these sorts of financing mechanisms is simple. Recently completed projects and possible nuclear designs are more expensive than alternatives, such as those that burn natural gas.
Also, with new capacity added in a few, very large increments and suppliers scattered throughout the world, it is difficult to build and sustain manufacturing and supply chain capability. That limits the ability to mass produce multiple units of the same design. Mass production is further hampered by the lack of an agreed international licensing process or “certification” for essentially duplicate designs, as adopted in and by the airline industry.
There is also a scarcity of global investment in R&D and demonstrations of completely new technologies, except in China, where literally “one-of-every-type” is being built and tested.
When the worldwide nuclear building plans are totaled, the numbers are promising: about 90 reactors and more than 90,000 MWe of net electrical output are slated to be built in the coming decade. Whether the nuclear power industry can meet this building schedule—or even keep the net number of operating reactors steady—remains to be seen. But the world needs even more.
Emerging Nuclear Tech
One potential way to break out of the current stasis is to develop a new generation of nuclear technology.
To be sure, many companies and organizations are working on concepts that are on a different scale, or use different fuel or alternative cooling media, or deviate in some other fundamental way from the light water reactors that dominate the industry at present.
Assessing the prospects of these concepts in not always easy. The key to the successful deployment of any such new or next-generation nuclear concept or design is the ability to compete against available energy alternates, especially, in local or national power markets.
The competition isn’t only the economics of supplying power, however, but rather holistic.
How should these proposals be assessed? This is not rocket science, but does require objective and rational thinking.
The requirements and internal challenges for any new nuclear concept, design, or technology can be summarized as follows:
• They should be safer than previous “generations” of nuclear technology;
• They should require a low financial risk exposure and capital cost;
• They should be easy to build quickly;
• They should be readily licensable by national authorities;
• They should be secure and simple to operate;
• They should have an assured and sustainable fuel supply;
• They should provide social value and acceptance;
• They should be competitive with respect to lowest cost generation.
One key factor that these requirements have in common is that they are quiet, if not exactly silent, on technological requirements. As long as a reactor concept can meet these eight challenges—meaning that it is “better” than existing nuclear power plants as well as gas turbines and coal thermal plants—it doesn’t matter what the fuel is or how it is cooled.
Further Reading: Harvesting the Power of the Ocean
It is the market that sets the needs and the requirements, and the exact design or means of achieving the breakthrough is secondary.
In any event, the reactor island is a small fraction of the total plant or project costs, so it is evident that technology choice is not crucial. The real issue is fully optimizing the overall cost and efficiency of the design and performance of any new technology to meet power- and financial-market requirements, not choosing or developing something that is superficially attractive, but too expensive.
Challenges and Solutions
For all the current advances in nuclear power, nuclear power plants have the following deficiencies: They generate radioactive wastes; they have relatively low thermal efficiencies, especially those plants equipped with water-cooled reactors; they risk radiation release during severe accidents; and they rely on nuclear fuel produced through environment-unfriendly processes. None of that is acceptable.
To expand the share of electricity produced by nuclear power—or to simply forestall a decline in nuclear’s “market share”—will require addressing those deficiencies as well as the eight requirements listed earlier.
A way to insure this is to establish the sales potential, target markets, and performance goals prior to committing to the preliminary design work. That can be done iteratively, by first assessing the optimum capital, operating, and generating costs as a function of plant output size to determine the system design targets and technical requirements. After that, one would need to establish investment needs and suitable power purchase agreements or contracts. Finally, one can determine the build profile and output that matches the power market and customer generating needs, iterating through the previous steps as needed, and if necessary changing or even adopting a different technology.
This systematic method provides a coherent business model for both supplier and customer, and is also useful as a rapid audit and estimating tool, and to weed out uncompetitive options.
Licensing, siting, fuel, and decommissioning costs are difficult to reduce substantially, so to meet the market needs, capital- and operating-cost reduction is the obvious first target. This argues against large plants, as recent build experience in China, Europe, and the United States shows that large plants often require more than 60 months to complete or experience significant delays in construction, and long schedules and delays are the major factor that must be avoided, since the levelized cost of energy grows almost linearly with the increase in project timescale.
Instead, the oft-stated objectives are to simplify and “modularize” designs, reducing capital and operating costs, and shortening construction times, perhaps building multiple smaller units instead of one large one to spread the risk and costs.
From Our Archives: Chernobyl 25 Years Later
The fundamental problem is that a decrease in plant output increases the levelized cost of energy, because many of the balance of plant and other variable and fixed costs (such as site, safety, infrastructure, engineering, decommissioning, and staffing) do not decrease proportionately, so ultimately become dominant as output shrinks. Also, unless there is a commitment to build a substantial number of these simplified, production-line or “Henry Ford” reactors, the engineering, design, licensing, and set-up costs all have to be absorbed by the first few units, increasing the cost of the first-of-its-kind unit far above the economic break-even point.
Instead, the nuclear industry could take a chapter from the aircraft manufacturing industry and use the “order book” approach, in which a company receives a commitment from customers for some set number of units of the same kind before it initiates a building program. To “fill the order book” is design and market specific, but the cost reduction possible from mass production is able to offset the penalty from building smaller units and plants.
(While both theory and real-world production data suggests that producing 10 or more units should decrease costs by up to 50 percent, it is now known that the actual AP1000 costs in the United States do not follow those projections, and neither do the current EPR builds in France and Finland, primarily due to long delays in construction and project schedule increasing the costs.)
From Our Archives: After Fukushima, ASME Task Force Challenges Nuclear Industry
Another, related concept is modular construction, which is widely used in oil rigs, military equipment, buildings, data centers, computers, and combined-cycle gas turbine installations. Rather than single large units, modules would be smaller in scale and could be added as needed to meet the local market demand for electricity.
Even the systems that make up the larger module can be “sub-modularized,” especially important when redundancy is needed for safety, maintenance, and performance reasons. The small modular reactor concept, or SMR, is predicated on each reactor unit being built in a factory of sorts and shipped to the site, rather than constructed on the spot.
Investment in module “factories” is expensive, requires large upfront commitment (for say, options for 100 standardized units in the “order book”), and the downside risks must be carefully managed, since that cost must also be subtracted, or amortized over the sale of many units. It is self-evident that although small units cost more for their power and energy, when mass produced they carry significantly less financial risk and for much shorter exposure times.
It is undeniable that SMRs are a very “hot” topic in nuclear engineering worldwide. Smaller or even “six pack” units could reduce investment risk, while adding capacity in increments to penetrate smaller electric grids and markets. According to the IAEA, there are about 55 SMRs designs or concepts proposed in the world. In 2019, Russia launched and licensed Akademik Lomonosov, a barge carrying its first two SMRs-KLT-40S reactors, intended as a floating nuclear power plant supplying 70 MW of electricity and heat for the country’s northern regions.
While the SMR concept is appealing, in fact, recent comparative studies by the Nuclear Energy Agency and the Nuclear Fuel Cycle Royal Commission in South Australia failed to demonstrate a clear market or price advantage for current SMR concepts, compared to competing nuclear technologies.
The challenges facing the nuclear industry as a whole—such as the low capital cost and high efficiency of modern natural gas and supercritical-pressure coal-fired power plants—will likely weigh down the development and deployment of SMRs in the near term.
Nuclear Plant of the Future
A simple comparison of recently published or deduced actual costs shows that presently built or proposed multi-unit nuclear plants (both large and small) must reduce specific capital and generating costs per kW by some 20 to 40 percent to compete with today’s market-ready combined-cycle gas turbines (CCGTs). This is not an easy target, and requires some radical rethinking of today’s developments—and discarding some concepts.
The next generation of nuclear power plants should be based on new designs—not just old ideas that have been rehashed yet again—to achieve the eight requirements. And these new designs must be better than “safe enough” or “safer than coal”; they should feature walk-away safety that precludes large accidents, core meltdown, and possible radiation releases, as well as the potential for weapons proliferation. The designs must produce electricity as cheaply as natural gas and with an efficiency in the range of CCGTs—better than 55 percent. Any new design must have broad public and regulatory acceptance and be capable of rapid licensing.
And it should go without saying that the next generation of nuclear reactors must provide for real, worldwide carbon-dioxide reduction without needing “carbon credits” or “offsets” to compete.
Further Reading: Startup Suns
For new nuclear power to contribute meaningfully to the fight against global warming requires a building program on a massive scale: Thousands of new units would need to come online, better than one per week from now until 2050. The industry is nowhere close to meeting that challenge, and policy makers nowhere near to accepting it—although the political realization is slowly growing that nuclear energy is necessary to the energy and environmental future of the planet.
The nuclear industry will need to take a hardheaded approach to get there. It must avoid fads and fantasies, as well as the lazy assertions that the world has no other options. It will require both the will to act and money in amounts large enough to turn the will into action. Nonprofit funding from groups such as the Gates Foundation is welcome, but not sufficient; it will require both private-sector and government investment.
Innovation is key, as is a willingness to reassess the assumptions of the past. Obviously, to meet the challenges set before the industry will require new directions in reactor design. But it also requires producing reactors that can match the needs of many different markets, both in scale and in cost. Small modular reactors (whatever that ultimately means) are one way to flexibly address the needs of markets, but it isn’t the only way, and it may not be appropriate for some markets. The tendency toward one-size-fits-all solutions is a poor one, regardless of the size.
To revive the nuclear-power sector will require not only a change in technology but also in mindset. It is too strong to say that the industry will have to start from scratch—after many decades, there is much to build on—but it is fair to say that a successful nuclear sector in 2050 will be radically transformed from what it is today.
Romney Duffey is former chair of ASME Nuclear Engineering Division and former principal scientist for Atomic Energy of Canada Limited. Igor Pioro is a professor of energy systems and nuclear science at the University of Ontario Institute of Technology in Oshawa. Pioro is the founding editor of the ASME Journal of Nuclear Engineering and Radiation Science.
This article is based on a JNERS paper from April 2019.