How Engineers Put Astronauts on the Moon
How Engineers Put Astronauts on the Moon
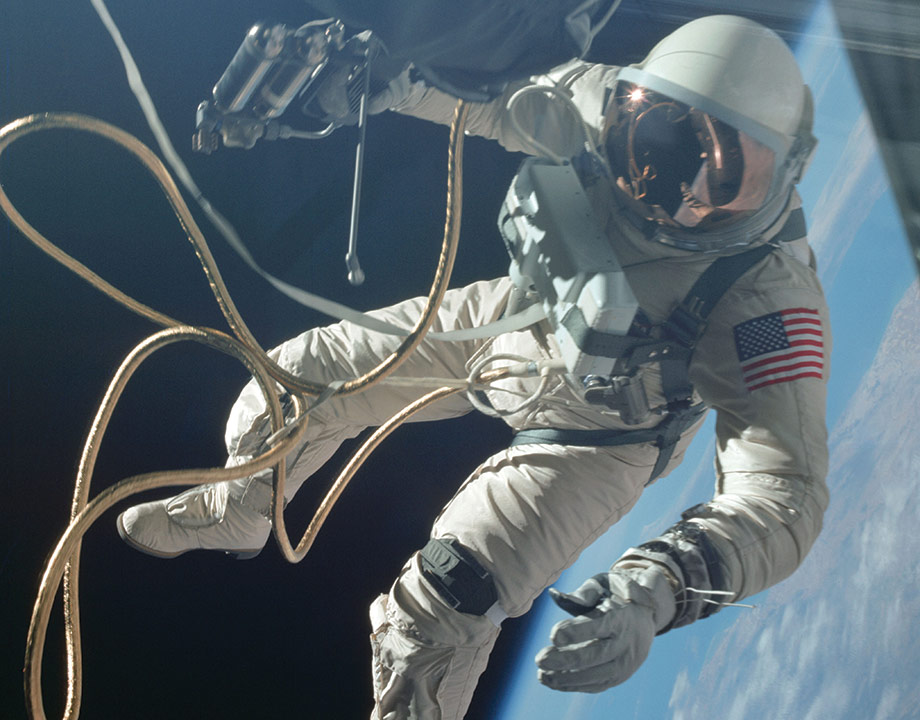
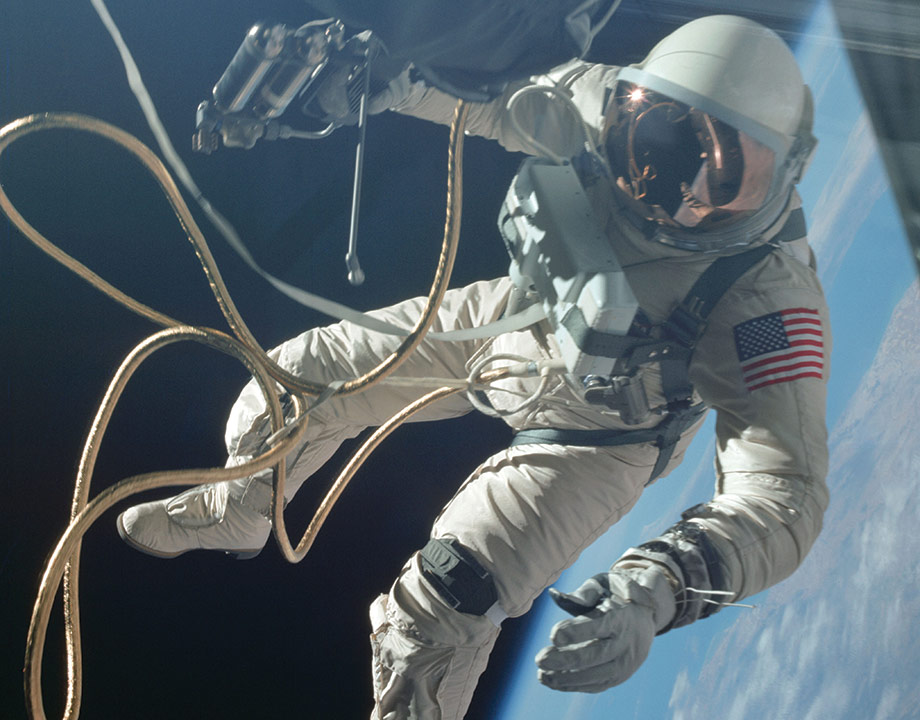
Astronaut Ed White conducted the first American extravehicular activity in 1965. NASA demanded extensive training and preparation before every mission. Photo credit: NASA
When the world heard President John F. Kennedy’s declaration that the United States would attempt to land astronauts on the Moon by the end of the decade, it was seen as a daring move by a bold, young leader. For NASA engineers like Robert Schwinghamer, a technical associate at the Marshall Space Flight Center in Huntsville, Ala., Kennedy’s May 1961 speech was more than that. It was a call to action.
At the time of Kennedy’s address to Congress, NASA was an organization less than three years old and had achieved only 15 minutes of human spaceflight experience—Alan Shepard’s suborbital flight in a small Mercury capsule. The agency had very little infrastructure or hardware and did not have a lunar landing in its immediate plans. NASA was now tasked with turning an aspirational idea into actual designs, procedures, and hardware.
The reality sank in fast that going to the Moon would require systems far more sophisticated than those used for Project Mercury. There were legitimate reasons to question the timeline Kennedy demanded. Some experts doubted it could be met—but not NASA’s young technical workforce.
“We were all young,” Schwinghamer recalled many years later. “We didn’t know what failure meant and we knew we could do it.”
Schwinghamer’s can-do attitude was pervasive throughout the fledgling space agency. Over the course of the next eight years leading up to the Apollo 11 lunar landing, engineers would confront hundreds of technical challenges.
History remembers Kennedy’s bold statement: “I believe that this nation should commit itself to achieving the goal, before this decade is out, of landing a man on the Moon and returning him safely to the Earth.” But a few moments later he stated, “In a very real sense, it will not be one man going to the Moon—if we make this judgment affirmatively, it will be an entire nation. For all of us must work to put him there.”
This is the story of some of that work.
Finding a Way
Only weeks after Kennedy’s speech, NASA awarded the first contract for the moonshot program—not for a rocket or spacecraft, but for the guidance and control system. The priority of that contract reflected the degree of challenge NASA expected in the design and construction of the system.
The contractor was the MIT Instrumentation Laboratory, a research arm of the university. The lab was headed by Charles Stark Draper, who had designed a revolutionary Inertial Guidance System used to navigate a B-29 bomber from Boston to Los Angeles in 1953. Inertial navigation quickly became commonplace in submarines, ballistic missiles, and aircraft.
NASA wanted this type of system for its Apollo spacecraft. The plan was to use a basketball-size inertial measurement unit (IMU) that would make use of three gyroscopes mounted perpendicular to each other to measure changes in direction and three accelerometers to measure changes in velocity.
While the IMU’s design challenged the lab’s engineers, the real unknown was how to build the digital computer the astronauts would use to interface with the IMU to receive and input information. In the early 1960s, even the most compact advanced digital computers were large enough to fill several rooms—much larger than the size of the proposed spaceship. When the engineers working on designing what would become the Apollo command module asked how much room to reserve for the computer, there was no immediate answer because such a computer had never been built.
Dick Battin, the lab’s technical director, conferred with his colleagues. Their estimate: “1 cubic foot.”
Then they had to build it to that spec.
The Apollo Guidance Computer (AGC) was one of the first to use integrated circuits, and engineers led by the lab’s deputy associate director, Hal Laning, came up with an elegant method for prioritizing computing tasks to speed up processing.
Even with that breakthrough, engineers were still unsure of the computer’s ability because of limited memory capacity and no firm grasp of the software needed.
The AGC that guided Apollo to the Moon had a memory capacity of only 72 kilobytes, mostly composed of copper wires woven in a specific pattern. Raytheon, which assembled the AGC, had a team of expert seamstresses who threaded the wires through magnetic rings (a wire going through the ring was a 1 and a wire going around the ring was a 0) to create the memory. Once this so-called rope memory was installed at the factory, however, it could not be changed.
Getting the programming right became the challenge, a task that was underestimated by engineers from both NASA and MIT. Margaret Hamilton, who led the team that would write the software for the AGC, summed up the reality of that development period. “When I first got into it, nobody knew what it was that we were doing. It was like the Wild West. There was no course in it. They didn’t teach it,” Hamilton said. In this vacuum, her team of more than 400 people not only developed the necessary programming for the AGC, they also essentially led to the creation of “software engineering” as its own discipline.
Listen to the latest episode of ASMETechCast: Engineering the Apollo 11 Lunar Module
Even so, the technology was unproven. NASA chose to use radio navigation to guide the Apollo spacecraft to the Moon with the AGC serving as backup. But the AGC would be the sole guidance system used during the critical phases when the spacecraft was on the far side of the Moon as well as to guide the lunar module to the Moon’s surface.
The big test occurred as Apollo 8 circled the Moon in December 1968 and re-emerged from the far side after being out of contact with Mission Control. A position check corresponded exactly with Earth-based calculations, verifying the AGC had the spacecraft exactly where it was supposed to be.
Notably, this first-of-its-kind computer never failed during any of the Apollo missions.
Design, Test, and Redesign
At the time of Kennedy’s address to Congress, rocket engine technology was starting to mature, but engineers faced a daunting challenge in developing a single system capable of lifting a 100,00 payload to the Moon.
Conceived by Wernher von Braun, the Saturn V started as a concept on a drawing board. As designed, it stood 363 feet tall and weighed 6.1 million pounds fully fueled. To lift it from the launch pad would require developing rocket engines 10 times more powerful than any U.S. rocket in existence. Each rocket would produce 1.5 million pounds of thrust and consume three tons of fuel and oxidizer every second; together, the five rockets making up the first stage of the Saturn V would propel the Apollo spacecraft to an altitude of 38 miles and a speed of Mach 7.
Engineers at NASA’s Marshall Space Flight Center and Rocketdyne, the prime contractor, now had to make it reality.
Some engineers confidently believed that developing the rocket engine, called the F-1, was simply a matter of scaling up existing engine designs—that the basics of rocket engines were the same. Early tests challenged that confidence when several engines exploded in the test stand.
Engineers traced the cause to “combustion instability,” a rotation of the flame inside the combustion chamber that could reach 2,000 cycles per second. The size of the engine was a contributing factor and there were no ready solutions. This led to questioning the feasibility of the engine. Donald Hornig, a member of the President’s Science Advisory Committee, lamented, “The engine might just be too big to make work.”
The entire Apollo program was in jeopardy.
Faced with this major challenge, the attitude of the engineers was crucial. “We had problems we did not know what to do about,” said James Murphy, the deputy manager of the Saturn V program. “It was the buildup of enthusiasm that made solving them possible.”
The investigation pointed to the injector, the showerhead-like mixer that fed the fuel and oxidizer through tiny holes into the combustion chamber. Initiating and sustaining combustion required the proper mixture ratio, pressure, and spray pattern. Once instability started, there was no way to stop it. It would destroy the engine in a matter of seconds.
The engineers experimented with enlarging the diameter of the orifices, adjusting the angles to control fuel and oxidizer impingement, and adding baffles on the injector plate to limit the rotational motion of the gases. In one series of tests, engineers placed small explosive devices inside the combustion chamber to create instability and measure the engine’s ability to recover.
There was no single engineering solution that fixed the problem. It took a meticulous approach that involved design, test, and redesign. After 18 months and thousands of engineering hours, including 78 hours of live engine testing, the F-1 engine was certified for flight on Sept. 6, 1966. The Saturn V flew 13 times during the Apollo program, and the F-1 engines achieved a 100 percent reliability rating.
Flexible and Functional
It would not be sufficient for astronauts to merely land on the Moon—they would need to explore. But the near vacuum of space and the temperature extremes on the lunar surface presented dangerous challenges. Astronauts would need a pressurized suit with a life-support system to provide oxygen and a comfortable temperature, as well as layered protection from the environment and micrometeoroids. In addition, the suit needed to be flexible enough to allow the astronauts to move around and perform tasks.
The silver spacesuit used by the astronauts on Project Mercury was an adapted Air Force high-altitude pressure suit. Walking on the Moon, however, would require something brand new. Like many other technological challenges for Apollo, the design requirements for such a suit were unknown.
In 1962, NASA invited companies to submit bids for the Apollo spacesuit. Eight companies entered the competition and the surprise winner was the International Latex Company (ILC), which proposed a simple design with flexible rubber joints. But ILC had no experience with space suits or working under a government contract—its most famous product was Playtex women’s undergarments. NASA appointed Hamilton Standard, a well-established aerospace supplier that had received the contract for the life support system, to oversee the entire program.
NASA required a very flexible suit that would enable the astronauts to stoop and bend in order to work on the Moon. In designing the suits, ILC made use of its talented pool seamstresses, who soon transitioned from women’s products to spacesuits. “I never thought in my wildest dreams I would be making space suits,” Eleanor Foraker, who managed the sewing operation, later declared. Foraker demanded the sewing quality meet the highest standards, as the astronauts’ lives were on the line.
Meanwhile, Hamilton Standard engineers were designing the Portable Life Support System (PLSS): The backpack that pressurized the suit, provided oxygen, removed carbon dioxide, and ensured a comfortable temperature. A main challenge was maximizing the oxygen capacity with minimal weight. Design engineer Earl Bahl had the assignment and, like other Apollo design tasks, he noted, “Nobody had done this before.” Bahl came up with a re-breathing system: Lithium hydroxide filters reacted with the exhaled CO2 to make lithium carbonate and water, while oxygen passed through the filter and was recycled. This reduced oxygen consumption by more than 20 percent.
In 1964, ILC and Hamilton Standard started to deliver finished suits. NASA rejected them because the suits were heavy, bulky, and not very flexible. The suits also failed a major test where astronauts had to fall onto their backs in the suit and stand back up.
In 1965, NASA took the drastic step of cancelling the contracts with ILC and Hamilton Standard and began an entire new competition. Starting from scratch was liberating for ILC, which proposed an innovative and very functional design based on its experience as a clothes designer. ILC again won the suit contract, this time reporting directly to NASA. (Hamilton Standard also retained the PLSS contract.) The new suit was three garments in one: A water-cooled undergarment; a pressurized inner suit that had flexible joints; and an outer nylon garment that protected against the temperature extremes and micrometeoroids. The suits, tested extensively, passed all of the requirements and were now ready for flight.
The Apollo 1 fire, which killed three astronauts during a ground test in January 1967, prompted another redesign. Following the accident investigation, NASA mandated the removal of all flammable material from the spacecraft and suit. ILC reached out to experts in fabric technology and found a material called beta cloth—tightly woven silica fiber coated in Teflon. With an outer shell made of beta cloth, the A7LB spacesuit was certified for flight.
Prepared for Any Contingency
The spacesuit delivered protection from the Moon’s harsh environment, but could the astronauts do meaningful work on the lunar surface? Project Gemini provided the proving ground to train astronauts for what NASA referred to as an extravehicular activity (EVA) and prepare them for the moonwalks.
Soviet cosmonaut Alexei Leonov completed the first EVA when he left his spacecraft for 12 minutes on March 18, 1965. The spacewalk was a great achievement for the Soviet program, but what was not reported at the time is that Leonov had to depressurize his spacesuit—a very dangerous procedure—in order to make the suit flexible enough to re-enter his capsule. It illustrated the unknowns of conducting EVAs.
Astronaut Ed White became the second spacewalker and the first for NASA when he exited his Gemini 4 spacecraft three months later. His 23-minute EVA proved the ability to exit and survive in space, but NASA quickly discovered that EVAs were not as easy as they might have looked. On NASA’s next EVA—during Gemini 9 in February 1966—astronaut Gene Cernan ran into trouble almost immediately. Cernan was tasked with assembling equipment outside the spacecraft, but without any handholds or foot restraints, Cernan mostly spun around as everything he pushed against pushed back. It was as if NASA had completely forgotten about Newton’s Third Law of Motion and Cernan got a refresher course.
The extra exertion sent Cernan’s heart rate to more than 170 and he quickly overtaxed his environmental cooling unit, which caused his visor to fog up. Cernan struggled for two hours before returning to the safety of the spacecraft. No meaningful work was accomplished and Cernan lost nearly 10 pounds in completing his dangerous EVA.
Following the flight NASA conducted an independent inquiry and found that planning and preparation for the EVA was severely deficient. It would not be until November 1966, the final flight in the Gemini program, that a successful EVA took place. Buzz Aldrin, who later became the second man to walk on the Moon, conducted extensive training in an underwater tank named the Neutral Buoyancy Trainer. Working with a mockup of the Gemini 12 spacecraft, in which the underwater environment simulated the zero-g experienced in space, Aldrin was able practice all the procedures.
Using installed handholds and footholds Aldrin conducted three spacewalks and was able to perform his entire task list without problems.
Editor’s Pick: Engineering Remember the Making of the Lunar Module
Training and practice in outdoor, indoor, and underwater environments became commonplace following Aldrin’s successful Gemini EVAs. By the time of Neil Armstrong’s “one small step,” he and Aldrin were prepared for any contingency on the lunar surface.
One might look back on the success of Apollo and believe it was pre-ordained. From a public view the 400,000 engineers, scientists, and technicians who contributed to the program made it seem easy. As these four Apollo technology examples illustrate, it was not.
The engineers and scientists who made Apollo possible never wavered. They displayed Robert Schwinghamer’s can-do attitude of “. . . We knew we could do it.” Because of their ingenuity, today we celebrate the 50th anniversary of Apollo 11 as a historic engineering and technological achievement.
Burton Dicht, an ASME Fellow, is the director of student and academic education programs at IEEE. His interest in aerospace history has made him a frequent lecturer on space-related topics.
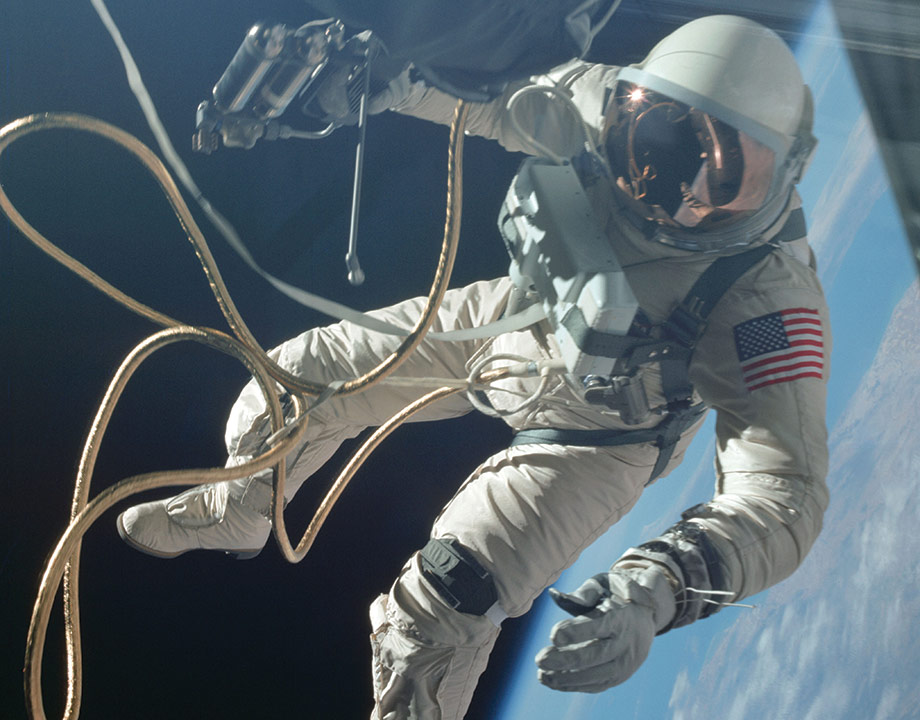
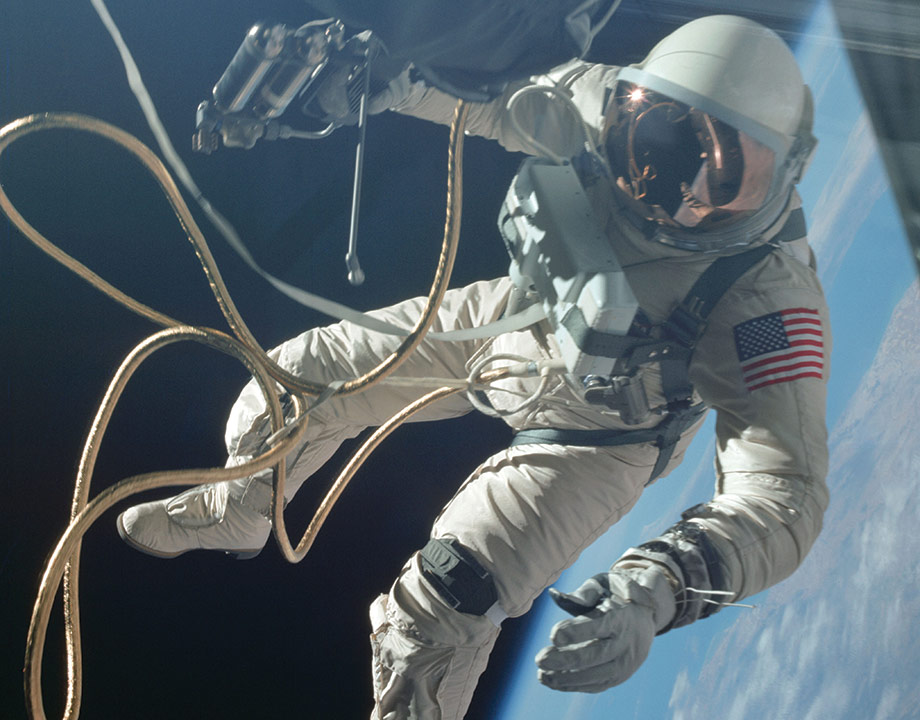