Designing Medical Implantable Devices with Multiphysics Simulation
Designing Medical Implantable Devices with Multiphysics Simulation
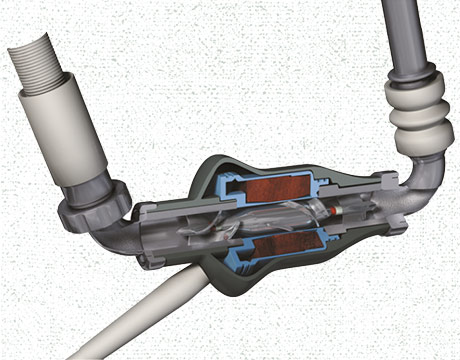
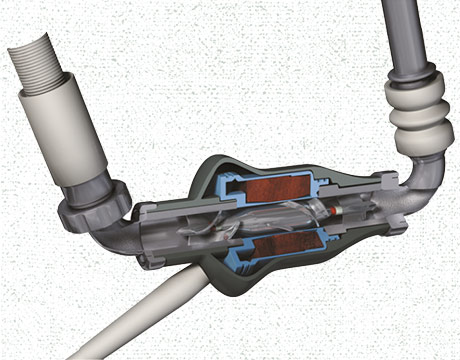
An LVAD pump is responsible for circulating oxygen-rich blood throughout the body. Image courtesy of St. Jude Medical (now Abbott). Photo courtesy: Thoratec Corporation
Case study provided by COMSOL
The development of a device meant to assist or completely replace functioning of the heart is undeniably complex. Researchers at St. Jude Medical (now Abbott) use multiphysics simulation to engineer Left Ventricular Assist Devices (LVADs), in an ongoing effort to improve the outlook and quality of life of patients with heart failure.
The design of an LVAD must take many factors into consideration. The device must be small enough to connect to the heart and be made of compatible materials and geometry that permit the device to reside in the body without being rejected. Fluid dynamics, power supply, and thermal management must also be considered. As multiple interacting physical effects must be accounted for at each area of development, multiphysics simulation is vital to the design process.
Freddy Hansen, Staff Research Physicist at St. Jude Medical (now Abbott) uses his expertise in physics and mathematical modeling to characterize complex implantable medical devices like LVADs before experimental studies. Hansen has created upwards of 230 models in COMSOL Multiphysics® that address a wide range of design challenges pertaining to the unique physics of artificial pumping devices.
“I use COMSOL Multiphysics every day, from proof of concept models to quite sophisticated simulations featuring detailed CAD geometries and coupled physics. I work with some complex models for months before I’ve taken all of the information I want from them.” Simulation allows for evaluating changes in size or geometry of the LVAD design before implementation of a physical prototype.
Optimizing LVAD Design
One challenge associated with engineering LVADs is the prevention of blood clotting in any space in or around the pump. To address this, a magnetically levitated rotor was developed, which eliminated the need for ball bearings and other components with geometries that might promote clotting. Hansen modeled both the magnetically levitated rotor and turbulent fluid flow.
The pump rotor receives blood axially and redirects it radially, into the volute, or fluid collector. Some of the blood flows back around the outer edge of the rotor and into the rotor inlet, resulting in a constant washing of the blood, which serves to eliminate places where the blood can stagnate and clot. Another significant advance was the development of a pump system with pulsatile flow, rather than continuous flow, which more closely mimics a functioning heart.
Wireless Powering
Current LVADs require power transfer from external batteries in a controller outside the body to the pump by way of a cable. But what if the cable could be eliminated? This would lead to a decreased infection risk and improved patient quality of life.
Hansen explored transferring power by way of magnetic resonance coupling that can be established even through a biological medium such as tissue. To assess the feasibility of wireless power transfer to an LVAD and determine how much power could be transferred between reasonably sized coils, Hansen coupled a 3D magnetic field model with an electrical circuit model to determine operating efficiency and power loss, as well as optimal circuit design and component values.
Engineers also had to ensure that body temperature and biological systems would not be affected by the implant. Hansen modeled the heat generated in the tissue as a result of the small currents induced by the wireless transfer of energy, combined this with models of heat generated inside the implant, and then used the thermal conductivity coefficient determined from a famous Cleveland Clinic experiment, to determine the temperature increase in body tissue near the implant.
Improved Options for Patients
In designing devices to assist and replace the function of the heart, multiphysics analysis has proven to be essential. Hansen combines experimental characterization and mathematical modeling to understand the physics pertaining to ventricle assist devices, and improve the biocompatibility of the device as well as the overall patient experience. The latest innovations to mechanical pumping systems hold much promise for better treatment in the future.
Learn more about the advances in modeling and simulation in Mechanical Engineering Magazine Special Report
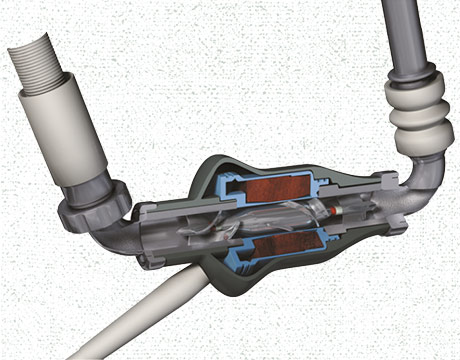
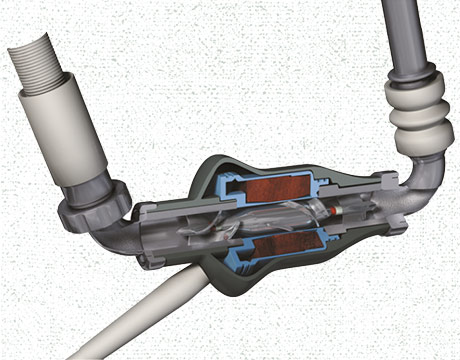