Carbon Reuse Turns a Cost into a Benefit
Carbon Reuse Turns a Cost into a Benefit
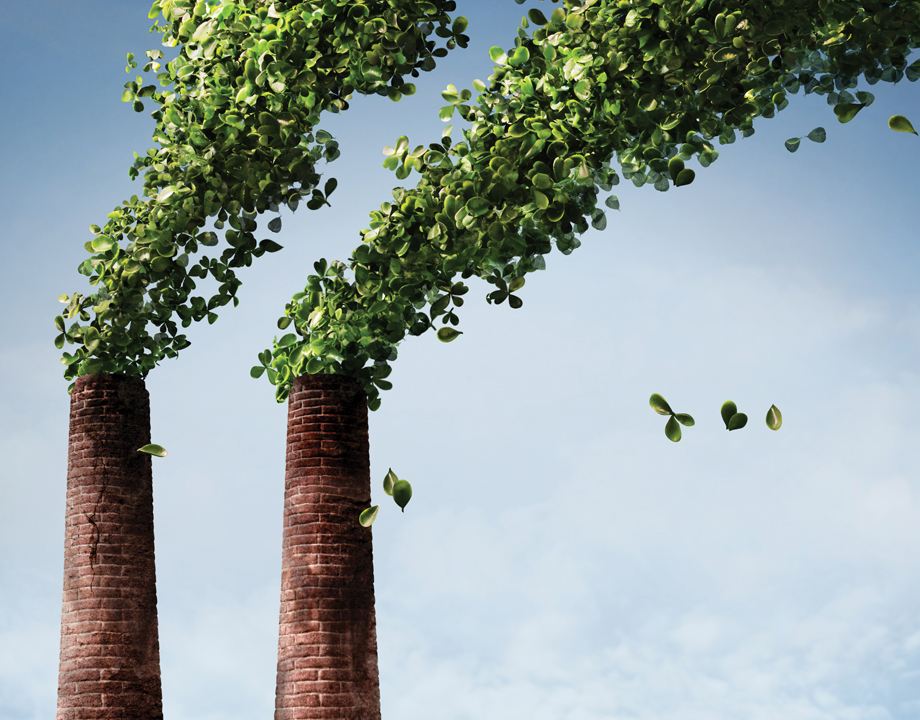
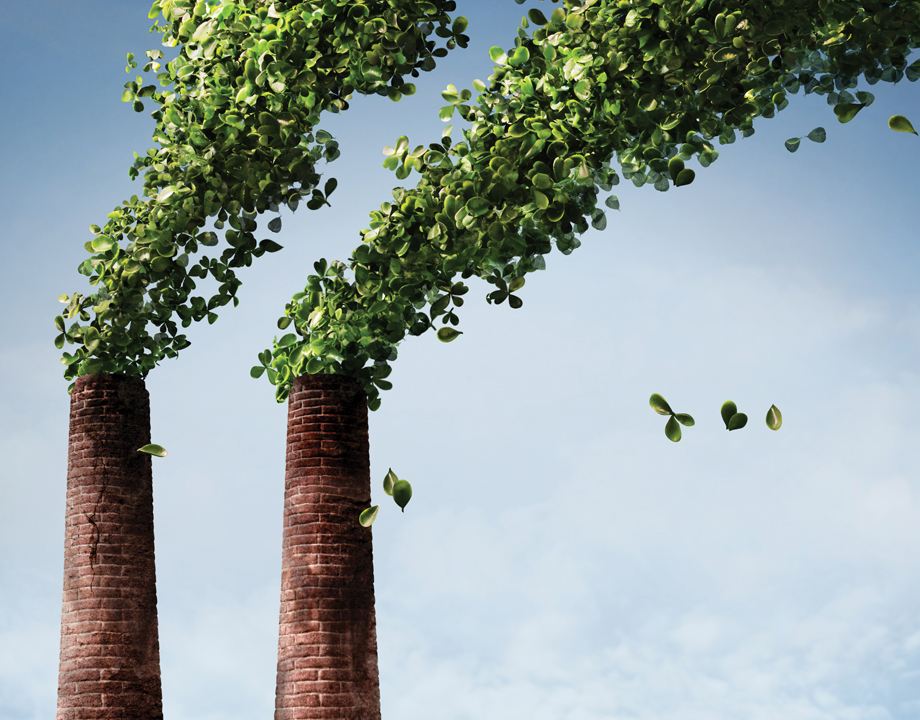
Industry has been dumping carbon dioxide into the atmosphere for more than a century. Now, entrepreneurs want to mine that excess carbon to make new products. Image: Getty
The natural cycle of carbon dioxide in the atmosphere is predictable. The concentration goes up in the Northern Hemisphere winter and goes down in the summer, tied to the annual growth of vast forests and grasslands. Over the course of a year, the fraction of CO2 in the atmosphere shows a peak-to-trough swing of 6 parts per million.
On top of that cycle there is a trend: The carbon dioxide that is emitted into the air by industries and other human activity at a rate faster than natural processes can clear it. From the pre-industrial average concentration of 280 parts per million, these activities have added another 135 ppm to bring the average in 2021 to around 415 ppm. That excess 135 ppm in the atmosphere equals just over 1 trillion tons of carbon dioxide, or a carbon mass of 288 billion tons.
All that excess carbon in the atmosphere is a problem—a crisis, actually. It is also an opportunity. Companies are looking at how to pull down that carbon and use it to make products.
“Carbon utilization is actually the holy grail of climate action if we can get it to work at scale,” said Peter Minor, a mechanical engineer who oversees innovation at Carbon180, a Washington, D.C.-based think tank. “Instead of thinking of emissions as pollution, we can turn them into a source of value. That completely changes the incentives around how we deal with CO2.”
Until now, actions to slow the rising concentration of carbon dioxide in the atmosphere have exacted a cost penalty. Wind and solar power were more expensive than coal, and methods of carbon capture were not only expensive, but also required a sequestration infrastructure to be built and maintained forever.
Regardless of the science of climate change, as well as the urgency, the market argued for the status quo.
Recently, though, the economics have begun to change. For instance, renewable power is now cheaper to build in many places than fossil power. If private companies can find a way to make profitable products from captured carbon, then free enterprise will leverage the marketplace toward climate action instead of against it.
While the technology of carbon capture and reuse is still very much in its early stages, the scope and breadth of innovation is truly breathtaking. Proposed uses have been emerging across a vast array of products and materials, whether in new ways to make traditional materials or in entirely new materials and products that are emerging into the space created by shifting priorities.
The potential resource is also vast. That 288 billion tons of excess carbon in the atmosphere? It is roughly the same as the world proven oil reserves, available for the taking from any point on Earth. Important changes in agriculture also show great promise as a means of transferring carbon from the atmosphere into the earth.
Getting at the excess carbon dioxide can be easy if you have time. Plants have been using photosynthesis to convert CO2 into solid mass for fuel and function for millions of years. Now, a growing number of companies are looking to replace carbon-intensive materials with biologically derived ones to lock up carbon for the lifetime of those products, potentially decades or longer.
For instance, small buildings such as single-family houses are made of carbon-storing wooden two-by-fours while larger multistory structures are built from carbon-intensive steel and concrete. Now, however, engineered wood products such as cross-laminated timber (CLT) and glulam produced by several companies are staking a claim to be negative-carbon alternative to steel and concrete in construction. CLT and glulam are structural beams made of layers of wood glued together with either parallel or perpendicular grain orientations.
More on This Topic: Building the Ultimate Carbon Capture Tree
Research has found that engineered wood is strong enough to support taller buildings than was previously thought possible. A recent lifecycle assessment study comparing two identical five-story building designs, one using wood and the other concrete for the frame, found that while the two buildings took the same amount of energy to build, the wood laminate building embodied 44 percent more captured carbon.
Other plant-based building materials include structural insulating panels made of compressed wheat straw by Agriboard of Vernon, Texas, and Willows, Calif.-based CalPlant’s fiberboard made from rice straw. JustBioFiber of Airdrie, Alberta, makes a hemp-based insulating structural block that’s far less expensive than concrete with a much lower carbon footprint. Similarly, several companies produce hempcrete, which contains hemp and mineral binders that absorb carbon during its curing process. It can be cast in place, purchased as blocks, or used as insulation.
There are carbon-negative opportunities indoors as well. Apart from traditional wood and plant-based furniture and textiles, Green Island, N.Y., startup Ecovative is making insulation and synthetic leather (as well as food and packaging) from processed mushroom mycelium. Flooring manufacturer Interface has reduced the carbon footprint of its carpet to the point whereby simply adding some bio-based materials to their backing, they are now selling the world’s first carbon-negative carpet tile.
Newlight Technology is going even further back in the evolutionary scale, while getting more high-tech. The Huntington Beach, Calif., company has spent the past decade developing a means to harvest polymers from bioreactor-grown microbes.
“In the ocean, there are microorganisms that eat both methane and carbon dioxide as their food source. In fact, these organisms played a key role in cleaning up the methane from the Gulf oil spill,” said Mark Herrema, Newlight’s CEO. “The organisms produce PHA (polyhydroxyalkanoates) in their cells for energy storage. We use a high-pressure filtration system to separate the biomass from the polymer. The polymer is then dried and extruded into pellets.”
An independent evaluation by Carbon Trust to calculate and certify the cradle-to-gate product carbon footprint of the polymer, marketed as AirCarbon, found that “for every kilogram of AirCarbon produced in Newlight’s production process, 88 kilograms of CO2e (obtained from methane seeping from abandoned coal mines) are sequestered.”
The company recently opened a 40,000-sq.-ft. factory to produce the ocean-degradable plastic for use in food ware, straws, eyeglass frames, and a leather-like material that can be used for wallets, phone cases, and other consumer products.
“It’s exciting to have a product made from greenhouse gas in people’s hands where they can see that carbon doesn’t have to go into the air,” Herrema said.
Other engineered systems skip biology altogether and start with physics and chemistry. Carbon dioxide gas is already a commercial product, created as a by-product of industrial processes, such as the conversion of methane and atmospheric nitrogen into ammonia or as the result of fermentation of corn into ethanol. It is sold for use in carbonated beverages or enhanced oil recovery. According to a recent market research report, the global carbon dioxide market was $9.68 billion in 2020.
Capturing that CO2 is good because it avoids venting it directly into the air. Drawing from the carbon reserve in the atmosphere requires developing a human-made method of harvesting it. Companies such as Carbon Engineering, Global Thermostat, and Climeworks have been working on direct-air capture machines for some time, but the technology is still expensive and inefficient.
The promise is great, however, as Klaus Lackner, a professor at Arizona State University in Tempe has said: “An artificial tree can collect carbon 1,000 faster than a real tree.” That’s a crucial factor when you consider the time scale needed, the fact that available land for tree-planting has numerous constraints, and the fact that those "trees" could potentially be mass-produced and then placed anywhere.
If direct-air capture is difficult and fast, the further challenge lies in what to do with that gas. For decades, the default has been to sequester it in geological formations. Using a different approach, Planetary Hydrogen in Ottawa electrolyzes seawater for hydrogen, but then adds a mineral salt to the electrolysis cell to make a mineral hydroxide. That chemical will absorb atmospheric CO2—as much as 40 kg for every 1 kg of hydrogen produced, the company says. It’s a speeded-up version of a naturally occurring process for scrubbing out atmospheric carbon.
More on Clean Energy: How Do We Zero Out Carbon?
If the electrolysis is powered by clean energy, the process results in carbon-negative hydrogen. In the end, though, the by-product would still need to be dumped. Finding a market for it would transform carbon capture from remediation—which is a cost to be borne by polluters or society—into a type of mining.
Since there’s only so much soda that people can drink, chemical companies are looking for new ways to convert carbon dioxide into products. For instance, Calgary-based Carbon Upcycling takes gaseous CO2 and embeds it into inorganic powders. “It’s a low-temperature batch adsorption process, produced in a rotating pressure vessel,” said Madison Savilow, the company’s chief of staff and the venture lead of its commercial arm, Expedition Air. Because the process does not require breaking the CO2 bond, she said, their process requires less energy than many others.
The company offers polyethylene, graphitic nanoplatelets, and talc with embedded CO2 content as high as 25 percent.
Other companies are also producing materials from recycled CO2 rather than other carbon feedstock. Richmond, Calif.-based Saratoga Energy uses a molten carbonate electrolysis process to convert carbon dioxide into carbon nanotubes and oxygen. According to the company, the process cuts costs dramatically and would have a negative carbon footprint once it is powered by renewable energy. German chemical company Covestro produces cardyon, a precursor material in the manufacture of polyurethane foam, via a process that uses CO2 as a feedstock; the material is up to 20 percent carbon dioxide.
Perhaps the biggest opportunity to utilize CO2 is in the building sector. Some 12 percent of human greenhouse gas emissions are the result of construction and the materials incorporated in buildings. Little wonder that builders are under pressure to reduce the carbon footprint embodied in buildings.
Another Perspective on Carbon Capture: Energy Blog: Trading One Crisis for Another
But the size of the challenge in the building sector also points to the magnitude of the opportunity. After all, due to their mass and relative permanence, buildings made from carbon-storing materials could lock up a great deal of carbon dioxide.
Concrete is likely the biggest opportunity for carbon utilization, since the construction industry uses so much of it. Some companies that are processing concrete in ways that absorb CO2 include Carbon Cure, Solidia, LafargeHolcim, and Blue Planet.
One recent development has been hybrid concrete. Wil Srubar, a civil engineer at the University of Colorado, has created a scaffold from sand and a nutrient-rich hydrogel that supported the growth of cyanobacteria. As the bacteria grow, they deposit the minerals that form “living materials” not unlike coral. Srubar’s research team said the material demonstrates strength similar to cement-based mortar. According to one press report, if you break one of these living bricks apart, the bacteria can use additional sand, hydrogel, and nutrients to essentially grow two complete bricks.
BioMason of Research Triangle Park, N.C., takes a similar approach to make a bio-cement from limestone grown in ambient temperature; the company sells a carbon-neutral, fully recyclable limestone tile that it claims is three times stronger than concrete block.
Realistically, though, such materials would be used as an offset for carbon-intensive processes. Chris Magwood of the Endeavour Center, a sustainable building school in Peterborough, Ontario, said that to achieve the net-zero target for which so many buildings are striving, “you need to balance the carbon-storing materials with those that inevitably have emissions.”
A major tool that has recently been developed to help builders with this is the Embodied Carbon in Construction Calculator (EC3) created jointly by the Carbon Leadership Forum and the international construction firm Skanska. The EC3 tool provides detailed information on the embodied carbon of more than 16,000 materials, including concrete, steel, wood, glass, aluminum, insulation, gypsum, carpet, and ceiling tiles, all based on environmental product declarations published by their manufacturers.
Before the EC3 tool, Magwood said, designers “would need to obtain lifecycle analysis data for each material selected, often relying on costly consultants. LCA is essential for understanding embodied carbon.” Now, this information is readily available.
“This is a journey,” said Stacy Smedley, director of sustainability at Skanska. “We’re probably on the second leg of a multi-leg journey when it comes to carbon-storing products in the building industry.”
Climate change is a problem of unprecedented scale and complexity. There is no single silver-bullet solution. The best chance of success will involve not simply walling off a set of climate actions, but incorporating solutions into every aspect of what we do, including buying everyday items. That train has already left the station and is gathering speed with each passing day.
The level of carbon dioxide in the atmosphere has been increasing relentlessly since the beginning of the Industrial Revolution, an unintended output of our economic engine. To bring those levels down, industry must find ways to use that waste stream as an input.
R.P. Siegel is a technology writer based in Rochester, N.Y.
On top of that cycle there is a trend: The carbon dioxide that is emitted into the air by industries and other human activity at a rate faster than natural processes can clear it. From the pre-industrial average concentration of 280 parts per million, these activities have added another 135 ppm to bring the average in 2021 to around 415 ppm. That excess 135 ppm in the atmosphere equals just over 1 trillion tons of carbon dioxide, or a carbon mass of 288 billion tons.
All that excess carbon in the atmosphere is a problem—a crisis, actually. It is also an opportunity. Companies are looking at how to pull down that carbon and use it to make products.
“Carbon utilization is actually the holy grail of climate action if we can get it to work at scale,” said Peter Minor, a mechanical engineer who oversees innovation at Carbon180, a Washington, D.C.-based think tank. “Instead of thinking of emissions as pollution, we can turn them into a source of value. That completely changes the incentives around how we deal with CO2.”
Until now, actions to slow the rising concentration of carbon dioxide in the atmosphere have exacted a cost penalty. Wind and solar power were more expensive than coal, and methods of carbon capture were not only expensive, but also required a sequestration infrastructure to be built and maintained forever.
Regardless of the science of climate change, as well as the urgency, the market argued for the status quo.
Recently, though, the economics have begun to change. For instance, renewable power is now cheaper to build in many places than fossil power. If private companies can find a way to make profitable products from captured carbon, then free enterprise will leverage the marketplace toward climate action instead of against it.
While the technology of carbon capture and reuse is still very much in its early stages, the scope and breadth of innovation is truly breathtaking. Proposed uses have been emerging across a vast array of products and materials, whether in new ways to make traditional materials or in entirely new materials and products that are emerging into the space created by shifting priorities.
The potential resource is also vast. That 288 billion tons of excess carbon in the atmosphere? It is roughly the same as the world proven oil reserves, available for the taking from any point on Earth. Important changes in agriculture also show great promise as a means of transferring carbon from the atmosphere into the earth.
Harnessing Nature
Getting at the excess carbon dioxide can be easy if you have time. Plants have been using photosynthesis to convert CO2 into solid mass for fuel and function for millions of years. Now, a growing number of companies are looking to replace carbon-intensive materials with biologically derived ones to lock up carbon for the lifetime of those products, potentially decades or longer.
For instance, small buildings such as single-family houses are made of carbon-storing wooden two-by-fours while larger multistory structures are built from carbon-intensive steel and concrete. Now, however, engineered wood products such as cross-laminated timber (CLT) and glulam produced by several companies are staking a claim to be negative-carbon alternative to steel and concrete in construction. CLT and glulam are structural beams made of layers of wood glued together with either parallel or perpendicular grain orientations.
More on This Topic: Building the Ultimate Carbon Capture Tree
Research has found that engineered wood is strong enough to support taller buildings than was previously thought possible. A recent lifecycle assessment study comparing two identical five-story building designs, one using wood and the other concrete for the frame, found that while the two buildings took the same amount of energy to build, the wood laminate building embodied 44 percent more captured carbon.
Other plant-based building materials include structural insulating panels made of compressed wheat straw by Agriboard of Vernon, Texas, and Willows, Calif.-based CalPlant’s fiberboard made from rice straw. JustBioFiber of Airdrie, Alberta, makes a hemp-based insulating structural block that’s far less expensive than concrete with a much lower carbon footprint. Similarly, several companies produce hempcrete, which contains hemp and mineral binders that absorb carbon during its curing process. It can be cast in place, purchased as blocks, or used as insulation.
There are carbon-negative opportunities indoors as well. Apart from traditional wood and plant-based furniture and textiles, Green Island, N.Y., startup Ecovative is making insulation and synthetic leather (as well as food and packaging) from processed mushroom mycelium. Flooring manufacturer Interface has reduced the carbon footprint of its carpet to the point whereby simply adding some bio-based materials to their backing, they are now selling the world’s first carbon-negative carpet tile.
Newlight Technology is going even further back in the evolutionary scale, while getting more high-tech. The Huntington Beach, Calif., company has spent the past decade developing a means to harvest polymers from bioreactor-grown microbes.
“In the ocean, there are microorganisms that eat both methane and carbon dioxide as their food source. In fact, these organisms played a key role in cleaning up the methane from the Gulf oil spill,” said Mark Herrema, Newlight’s CEO. “The organisms produce PHA (polyhydroxyalkanoates) in their cells for energy storage. We use a high-pressure filtration system to separate the biomass from the polymer. The polymer is then dried and extruded into pellets.”
An independent evaluation by Carbon Trust to calculate and certify the cradle-to-gate product carbon footprint of the polymer, marketed as AirCarbon, found that “for every kilogram of AirCarbon produced in Newlight’s production process, 88 kilograms of CO2e (obtained from methane seeping from abandoned coal mines) are sequestered.”
The company recently opened a 40,000-sq.-ft. factory to produce the ocean-degradable plastic for use in food ware, straws, eyeglass frames, and a leather-like material that can be used for wallets, phone cases, and other consumer products.
“It’s exciting to have a product made from greenhouse gas in people’s hands where they can see that carbon doesn’t have to go into the air,” Herrema said.
Chemical Solution
Other engineered systems skip biology altogether and start with physics and chemistry. Carbon dioxide gas is already a commercial product, created as a by-product of industrial processes, such as the conversion of methane and atmospheric nitrogen into ammonia or as the result of fermentation of corn into ethanol. It is sold for use in carbonated beverages or enhanced oil recovery. According to a recent market research report, the global carbon dioxide market was $9.68 billion in 2020.
Capturing that CO2 is good because it avoids venting it directly into the air. Drawing from the carbon reserve in the atmosphere requires developing a human-made method of harvesting it. Companies such as Carbon Engineering, Global Thermostat, and Climeworks have been working on direct-air capture machines for some time, but the technology is still expensive and inefficient.
The promise is great, however, as Klaus Lackner, a professor at Arizona State University in Tempe has said: “An artificial tree can collect carbon 1,000 faster than a real tree.” That’s a crucial factor when you consider the time scale needed, the fact that available land for tree-planting has numerous constraints, and the fact that those "trees" could potentially be mass-produced and then placed anywhere.
If direct-air capture is difficult and fast, the further challenge lies in what to do with that gas. For decades, the default has been to sequester it in geological formations. Using a different approach, Planetary Hydrogen in Ottawa electrolyzes seawater for hydrogen, but then adds a mineral salt to the electrolysis cell to make a mineral hydroxide. That chemical will absorb atmospheric CO2—as much as 40 kg for every 1 kg of hydrogen produced, the company says. It’s a speeded-up version of a naturally occurring process for scrubbing out atmospheric carbon.
More on Clean Energy: How Do We Zero Out Carbon?
If the electrolysis is powered by clean energy, the process results in carbon-negative hydrogen. In the end, though, the by-product would still need to be dumped. Finding a market for it would transform carbon capture from remediation—which is a cost to be borne by polluters or society—into a type of mining.
Since there’s only so much soda that people can drink, chemical companies are looking for new ways to convert carbon dioxide into products. For instance, Calgary-based Carbon Upcycling takes gaseous CO2 and embeds it into inorganic powders. “It’s a low-temperature batch adsorption process, produced in a rotating pressure vessel,” said Madison Savilow, the company’s chief of staff and the venture lead of its commercial arm, Expedition Air. Because the process does not require breaking the CO2 bond, she said, their process requires less energy than many others.
The company offers polyethylene, graphitic nanoplatelets, and talc with embedded CO2 content as high as 25 percent.
Other companies are also producing materials from recycled CO2 rather than other carbon feedstock. Richmond, Calif.-based Saratoga Energy uses a molten carbonate electrolysis process to convert carbon dioxide into carbon nanotubes and oxygen. According to the company, the process cuts costs dramatically and would have a negative carbon footprint once it is powered by renewable energy. German chemical company Covestro produces cardyon, a precursor material in the manufacture of polyurethane foam, via a process that uses CO2 as a feedstock; the material is up to 20 percent carbon dioxide.
Perhaps the biggest opportunity to utilize CO2 is in the building sector. Some 12 percent of human greenhouse gas emissions are the result of construction and the materials incorporated in buildings. Little wonder that builders are under pressure to reduce the carbon footprint embodied in buildings.
Another Perspective on Carbon Capture: Energy Blog: Trading One Crisis for Another
But the size of the challenge in the building sector also points to the magnitude of the opportunity. After all, due to their mass and relative permanence, buildings made from carbon-storing materials could lock up a great deal of carbon dioxide.
Concrete is likely the biggest opportunity for carbon utilization, since the construction industry uses so much of it. Some companies that are processing concrete in ways that absorb CO2 include Carbon Cure, Solidia, LafargeHolcim, and Blue Planet.
One recent development has been hybrid concrete. Wil Srubar, a civil engineer at the University of Colorado, has created a scaffold from sand and a nutrient-rich hydrogel that supported the growth of cyanobacteria. As the bacteria grow, they deposit the minerals that form “living materials” not unlike coral. Srubar’s research team said the material demonstrates strength similar to cement-based mortar. According to one press report, if you break one of these living bricks apart, the bacteria can use additional sand, hydrogel, and nutrients to essentially grow two complete bricks.
BioMason of Research Triangle Park, N.C., takes a similar approach to make a bio-cement from limestone grown in ambient temperature; the company sells a carbon-neutral, fully recyclable limestone tile that it claims is three times stronger than concrete block.
Realistically, though, such materials would be used as an offset for carbon-intensive processes. Chris Magwood of the Endeavour Center, a sustainable building school in Peterborough, Ontario, said that to achieve the net-zero target for which so many buildings are striving, “you need to balance the carbon-storing materials with those that inevitably have emissions.”
A major tool that has recently been developed to help builders with this is the Embodied Carbon in Construction Calculator (EC3) created jointly by the Carbon Leadership Forum and the international construction firm Skanska. The EC3 tool provides detailed information on the embodied carbon of more than 16,000 materials, including concrete, steel, wood, glass, aluminum, insulation, gypsum, carpet, and ceiling tiles, all based on environmental product declarations published by their manufacturers.
Before the EC3 tool, Magwood said, designers “would need to obtain lifecycle analysis data for each material selected, often relying on costly consultants. LCA is essential for understanding embodied carbon.” Now, this information is readily available.
“This is a journey,” said Stacy Smedley, director of sustainability at Skanska. “We’re probably on the second leg of a multi-leg journey when it comes to carbon-storing products in the building industry.”
Climate change is a problem of unprecedented scale and complexity. There is no single silver-bullet solution. The best chance of success will involve not simply walling off a set of climate actions, but incorporating solutions into every aspect of what we do, including buying everyday items. That train has already left the station and is gathering speed with each passing day.
The level of carbon dioxide in the atmosphere has been increasing relentlessly since the beginning of the Industrial Revolution, an unintended output of our economic engine. To bring those levels down, industry must find ways to use that waste stream as an input.
R.P. Siegel is a technology writer based in Rochester, N.Y.
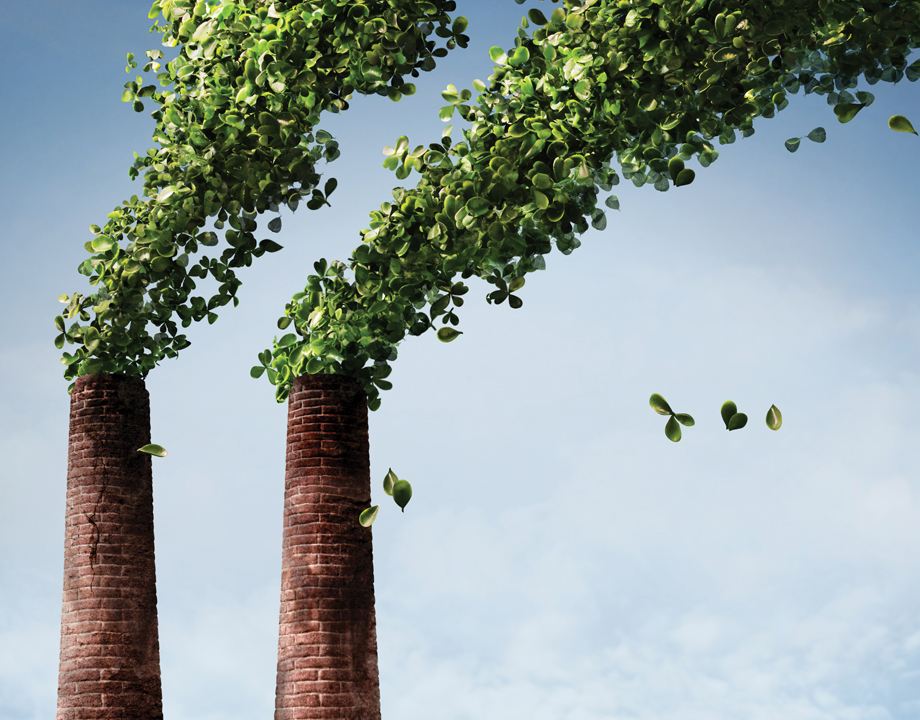
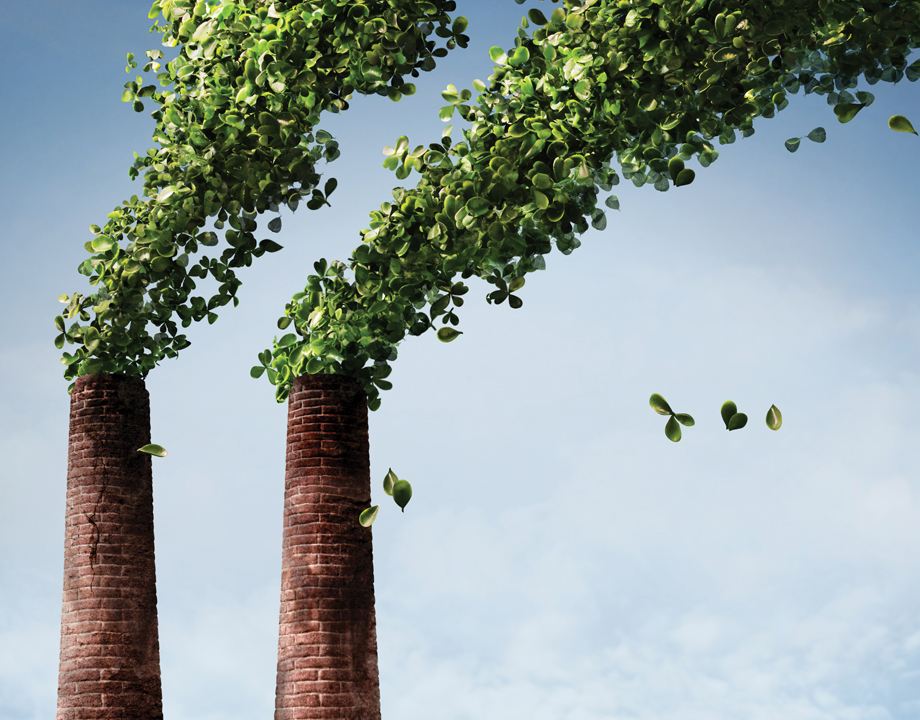