Advancing Battery Technology for Modern Innovations
Advancing Battery Technology for Modern Innovations
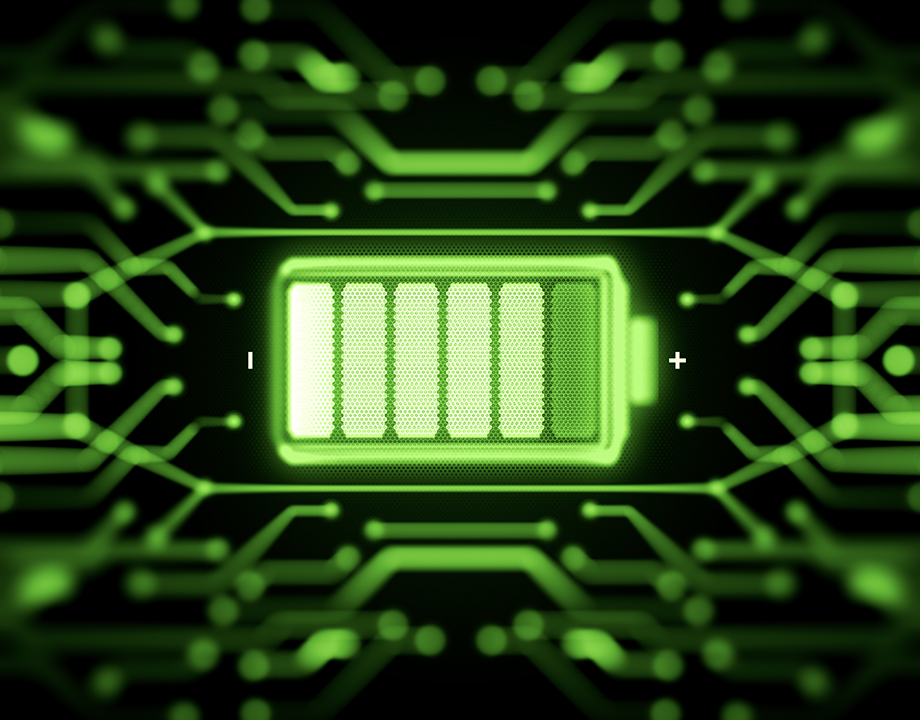
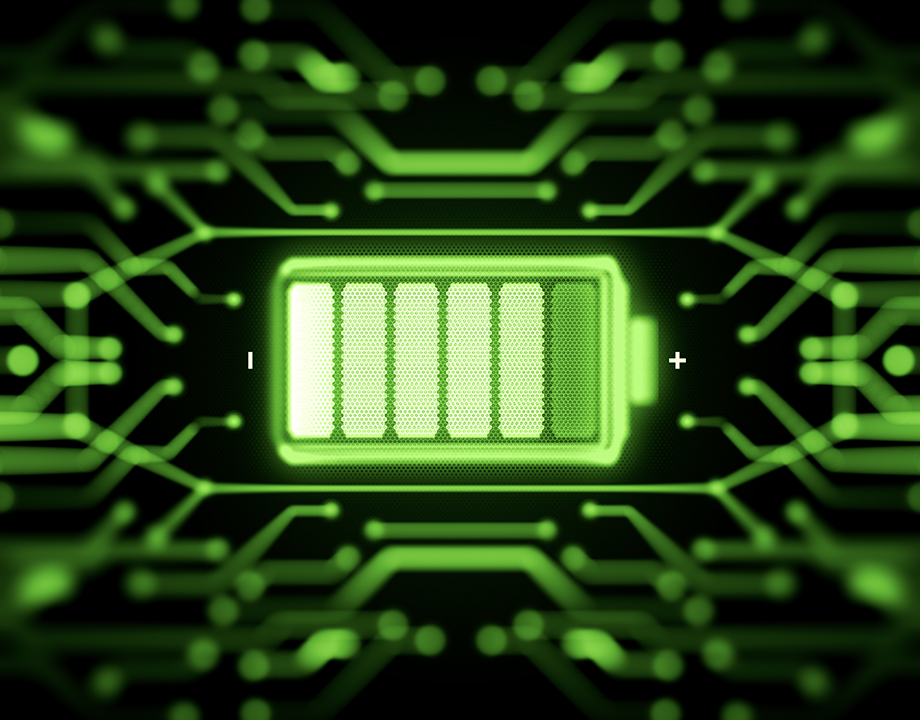
Advances in mobile devices and electric vehicles have pushed battery technology to the breaking point. New advances in battery design are needed to meet today’s energy demands.
This story was updated on 2/1/2023.
Batteries have always been a critical design feature, for everything from handheld tools to computers and mobile phones and from uninterruptible power supplies to satellites. Battery research has been going on for years to increase energy density (the amount of energy in a given size and weight)—the need of which came during the upswing in handheld devices, from industrial measuring tools to mobile phones.
The increase in telecommunications satellites meant that battery weight was a factor, and every technological advancement tended to put battery capabilities in the forefront. While laboratories were working on upgrading battery technology, electronics technologies continued to advance more quickly—requiring ever-increasing amounts of energy and power.
But it wasn’t until electric vehicles (EVs) came onto the scene that manufacturers began to seriously consider the importance of batteries to provide greater range, higher reliability, and lower costs. For the EV market, size and weight are as important as cycle life. Categorized as primary (single-use typically for long-term, low power applications) and secondary (rechargeable), batteries have seen one innovation after another as they attempt to provide more energy density than ever before.
The Current State of Batteries
Today, state-of-the-art primary battery technology is based on lithium metal, thionyl chloride (Li-SOCl2), and manganese oxide (Li-MnO2). They are suitable for long-term applications of five to twenty years, including metering, electronic toll collection, tracking, and the Internet of Things (IoT). The leading chemistry for rechargeable batteries used in telecom, aviation, and rail applications is nickel-based (Ni-Cd, Ni-MH) batteries. Lithium-based (Li-ion) batteries dominate the consumer electronics market and have expanded their applications to electric vehicles.
It’s important to note here that the quantity of Li-ion batteries used in EVs exceeds the volume of mobile and IT applications combined.
Lithium-ion batteries, spurred by the growth in mobile phone, tablet, and laptop computer markets, have been pushed to achieve increasingly higher energy densities, which are directly related to the number of hours a battery can operate. Battery experts in the field have continually adjusted the technology to gain greater densities, including changing chemistries and modifying the designs. They’ve even looked at the raw material supply chain, considering it expensive and difficult to source cobalt as an additive to Li-ion designs.
Energy density is measured in Watt-hours per kilogram (Wh/kg). Li-ion designs provide the highest density of up to 250-270 Wh/kg for commercially available batteries. As a comparison, consider that lead-acid batteries offer less than 100 Wh/kg and nickel metal hydride batteries reach barely over 100 Wh/kg.
In addition to energy density, power density is also an important consideration. Power density measures the rate a battery can be discharged (or charged) versus energy density, which is a measure of the total amount of charge. A high-power battery, for example, can be discharged in just a few minutes compared to a high-energy battery that discharges in hours. Battery design inherently trades energy density for power density.
“Li-ion batteries can be extremely powerful in terms of power density,” says Joong Sun Park, technical manager for Solid State Technology. “Saft produces one of the highest power density Li-ion cells in the world used in Joint Strike Fighter and Formula 1 racing cells that range up to 50kW/kg.”
Li-ion battery technology has progressed significantly over the last 30 years, but the best Li-ion batteries are nearing their performance limits due to material limitations. They also have significant safety concerns—such as catching on fire if overheated—leading to increased costs because safety features must be designed into the battery system.
Recommended for You: Developing Fast-Charging Batteries
“There are alternative materials and battery chemistry being developed to go beyond Li-ion including lithium-sulfur, sodium, magnesium (Li/S, Na, Mg)-based designs,” said Park, regarding alternative materials to lithium ion. “These certainly have potential benefits over existing Li-ion batteries in terms of energy density or cost once commercialized. However, the maturity of technology is still low compared with Li-ion at the moment. Therefore, further breakthroughs from usable materials to manufacturing are required to compete with Li-ion.”
Ultimately, it appears that Li/S batteries are not ready for commercialization due to gaps between practical production and academic research at this time but are seriously being researched.
“The push to decrease the carbon footprint also drives the development of sustainable energy generation like solar and wind, combined with a storage device, such as a battery,” said Park.
This alludes to the fact that greater demands lead to the innovation of material selection, design, and manufacturing processes. Materials such as solid polymer, ceramic, and glass electrolyte enable solid-state batteries and new environmentally benign processes to remove the use of toxic solvents that are used during the manufacturing processes of Li-ion batteries.
Solid-State Batteries
Although the current industry is focused on lithium-ion, there is a shift into solid-state battery design.
“Lithium-ion, having been first invented and commercialized in the 90s, has, by and large, stayed the same,” said Doug Campbell, CEO and co-founder of Solid Power, Inc. “You pretty much have the same electrode combinations with some minor tweaks. The industry has engineered about as much as they can out of the technology.”
Solid Power has experimented with several types of materials including polymer, oxide, and sulfide. Each has its benefits and drawbacks. Through their research, they’ve chosen to develop sulfide technology further.
Test Your Knowledge: What Do You Know About Rural Electrification?
Moving from a liquid electrolyte battery to a solid-state battery might appear to be outside the conventional design, but it’s aimed at leapfrogging present capabilities in energy density. Metallic lithium forms dendrites in a liquid battery system, which compromise cycle life and the batteries’ safety. Replacing the highly reactive liquid electrolyte with a solid-state electrolyte, which is inherently safer and mechanically more rigid, increases the battery’s energy density without compromising safety.
Solid-state battery technology incorporates solid metal electrodes as well as a solid electrolyte. Although the chemistry is generally the same, solid-state designs avoid leakage and corrosion at the electrodes, which reduces the risk of fire and lowers design costs because it eliminates the need for safety features. The solid electrolyte design also allows for a smaller form factor, meaning less weight. Most importantly, solid-state batteries are expected to overcome the current limitations of energy density. It is believed that using metallic lithium will, theoretically, double the capacity of the Li-ion cell technology if adequately designed. Metal lithium has a ten times higher capacity than standard carbon anodes used in current Li-ion batteries.
Why Shift to Solid-State Batteries?
The industry is presently shifting to solid-state batteries for multiple reasons.
The foremost is that standard lithium batteries with a liquid electrolyte have bumped up against the theoretical limits of the electrode combinations being used, even when fine-tuning the design to gain more density.
Yet, from a market standpoint, with EVs coming on strong in the market, there is a significant call for ever-increasing energy densities—where every increase is directly proportional to increased vehicle range and battery life in general. The need for much higher capacity electrodes, such as solid lithium metal, means you’re looking at anywhere from 50 to 100 percent improvements in Watt-hour per kilogram. Plus, some ancillary benefits include replacing the volatile and flammable liquid electrolyte with a stable and solid material that will not exhibit the thermal runaway problem seen in the past—for example, how solid lithium-ion is a much safer chemistry.
However, there are still issues to resolve, such as which materials are the most efficient and which production techniques yield the lowest end-product cost. Presently, the solid-state batteries that are able to compete in the market are limited to small cells. The first commercially available solid-state batteries are thin-film batteries, which are nano-sized batteries composed of layered materials that function as electrodes and electrolytes. Thin-film solid-state batteries resemble, in structure, conventional rechargeable batteries except that they are very thin and flexible. Besides lighter weight and small size, thin-film batteries offer higher energy density for smaller electronic devices such as pacemakers, wireless sensors, smart cards, and RFID tags.
Besides resolving the issues of affordability and scale, solid-state batteries also have technological challenges. While solid-state batteries are much safer, there is still the matter of dendrites—the root-like build-up that happens on lithium metal in the anodes that form as the battery charges and discharges. Dendrite build-up reduces the amount of solid electrolyte capacity and thus the stored charge.
Finding the right separator material that allows lithium ions to flow between the electrodes—while also blocking dendrites—is the greatest challenge for developers. According to the paper Interface Stability in Solid-State Batteries, researchers have used materials such as a polymer, which is widely used in liquid electrolyte batteries, or a hard ceramic. The polymer doesn’t block dendrites, and most ceramics are brittle and don’t last for multiple charging cycles. Once the dendrite problem is solved, solid-state batteries are expected to offer consumers some enticing performance advantages: faster charging, higher energy density, longer life cycle, and greater safety.
Another method being developed is an anode-free design. When the battery discharges during use, the lithium flows from the anode to the cathode. In this case, the anode reduces in thickness. This process is reversed when the battery is charged and the lithium ions flood back into the anode.
You May Also Like: How the Food Web Can Keep the Electricity Flowing
Another company, Sion Power, has moved from Li/S to their Licerion Lithium-Metal Technology. According to their technical information, Sion Power overcame the issues that plagued historical lithium metal chemistries—energy density (Wh/L) and cycle life—by developing a multi-faceted approach to protecting the lithium metal anode. They incorporate three levels of protection: chemical protection within the cell, physical protection within the cell, and physical protection at the pack level. They use a patented protected lithium anode (PLA) technology whereby the lithium metal anode is physically protected by a thin, chemically stable, and ionically conductive ceramic polymer barrier.
This allows the electrolyte additives at the cell level to stabilize the anode surface, which enhances cycle life and increases energy. The pack incorporates proprietary cell compression and an advanced battery management system as well.
The Future of Energy Storage
The race is on. With EV sales skyrocketing, the need for high-density, long life, and low-cost batteries means the competitive landscape for solid-state batteries is becoming crowded. This is great news for the research and development of these batteries since that’s what’s needed to get solid-state batteries onto the market quickly. At this point in time, several materials and designs are being explored and showing significant progress.
With small cells already proving the higher capabilities needed from solid-state batteries, it’s only a matter of time before the manufacturing processes fall into place for larger batteries as well. Several companies have suggested that we’ll see these batteries on the market within the next few years. Once manufacturing catches up, just like liquid electrolyte Li-ion batteries, technological innovations will push us further. This means that we’re likely to see tweaking of materials and design approaches that will push battery capabilities forward for years to come.
Terry Persun is a technology writer based in Port Townsend, Wash.